Ultimate Power: Synthetic Pathogens & Designer Genetic Bioweapons
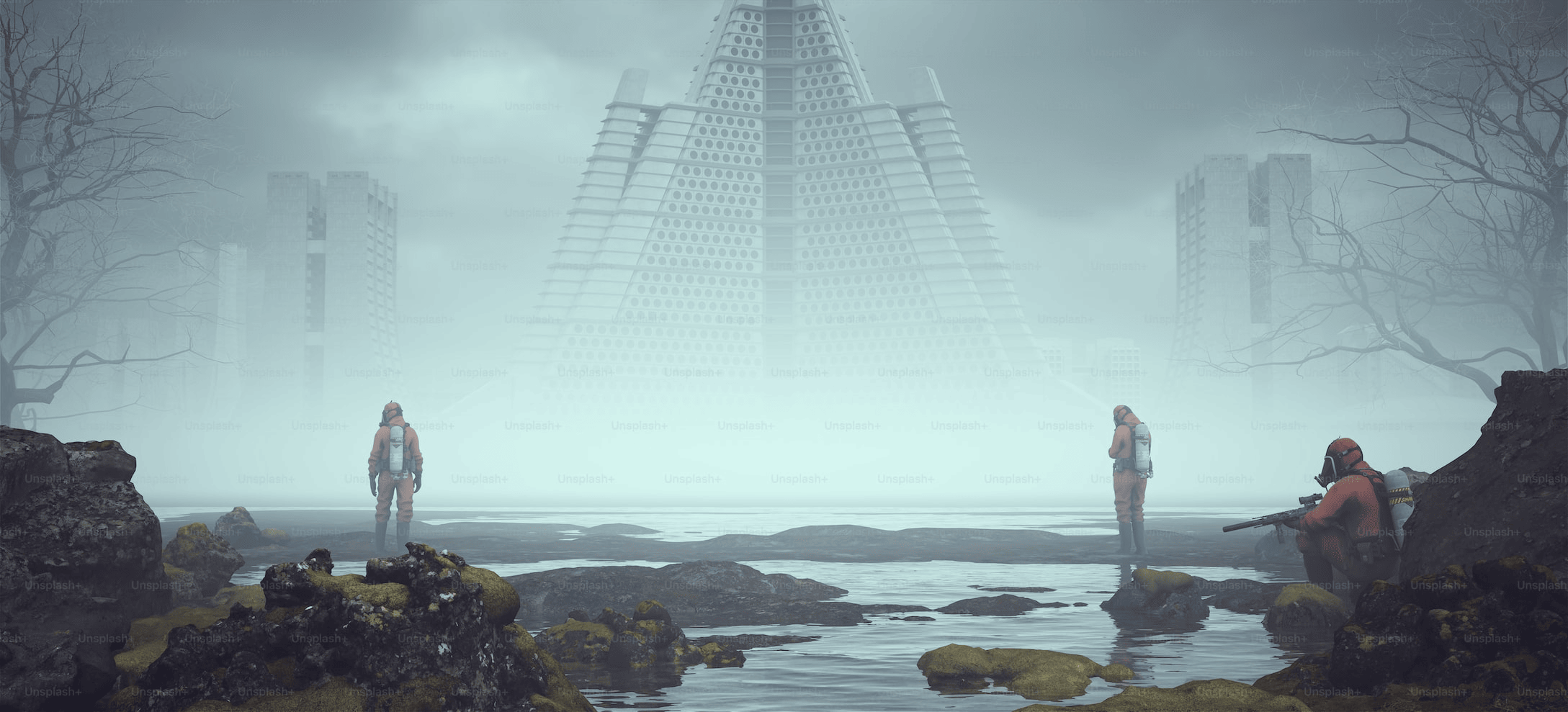
Post-apocalyptic fiction based on the biological destruction of humankind is a common theme, but how plausible are genetic superweapons (SBWs) wielded by supervillains? Alarmingly, they've been the next frontier in WMDs since the early nineties. We have no idea how far military scientists have got in their R&D, if at all. But military agencies have been extremely worried for a long time, that it is only a matter of time.
How real is this? Read for yourself:
- https://en.wikipedia.org/wiki/Ethnic_bioweapon
- https://www.ncbi.nlm.nih.gov/pmc/articles/PMC1326447/
- https://carnegieendowment.org/2018/04/17/new-killer-pathogens-countering-coming-bioweapons-threat-pub-76009
- https://sites.dartmouth.edu/dujs/2013/03/10/genetically-engineered-bioweapons-a-new-breed-of-weapons-for-modern-warfare/
- https://thebulletin.org/premium/2020-12/how-to-protect-the-world-from-ultra-targeted-biological-weapons/
- https://www.usni.org/magazines/proceedings/2021/june/synthetic-bioweapons-are-coming
Is There Anything Worse Than A Nuke?
A nuclear bomb causes immediate, widespread destruction through blast, heat, and radiation. The blast and heat can instantly kill thousands or even millions of people, depending on the size of the bomb and the population density of the target area. Additionally, nuclear weapons release ionizing radiation that can cause acute radiation sickness, long-term health problems, and environmental contamination, rendering affected areas uninhabitable for long periods.
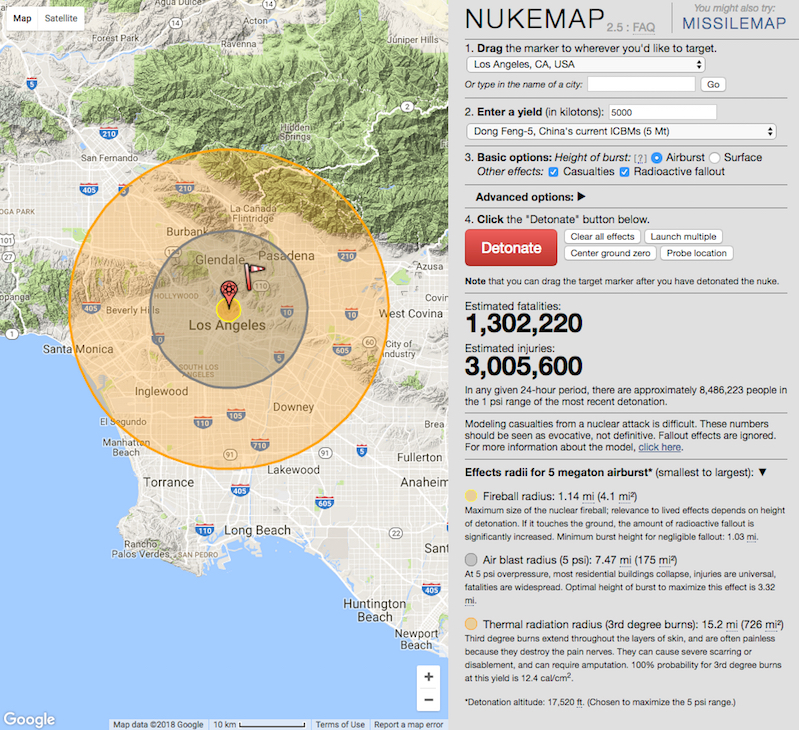
For example, if a thermonuclear warhead, perhaps a 1 megaton thermonuclear bomb (which is roughly 50 times more powerful than the bomb dropped on Hiroshima), and a ground burst detonation in downtown Los Angeles, population 4M - as quite a few people would definitely like it to be - the results would be catastrophic:
- The immediate area around the detonation point (within a radius of approximately 1-2 miles) would be completely obliterated by the blast. Nearly everyone would be killed instantly. This could result in hundreds of thousands of fatalities.
- Beyond the blast zone, the heat from the explosion would cause severe burns and start fires potentially miles away from ground zero.
- People close to the blast might suffer from acute radiation syndrome, while others further away could be at a higher risk of developing cancer and other diseases in the future.
But....
A genetic weapon, on the other hand, would cause harm in a more targeted and insidious manner. The engineered virus could spread silently and rapidly through a population, infecting specific individuals based on their genetic makeup. The effects of the genetic weapon would depend on the custom gene sequence and the intended harm, which could range from debilitating illness to rapid or slow death. The damage caused by a genetic weapon might not be as immediately visible as that of a nuclear bomb, but it could still lead to widespread suffering and loss of life. Furthermore, the psychological impact of a genetic weapon, particularly one targeting specific ethnic or demographic groups, could be severe and long-lasting.
Put simply, there is no more terrifying, planet-destroying horror than a genetic bioweapon. A nuke would kill millions in an instant, but a synthetic pathogen would kill tens of millions across an entire continent - taking the economy with it, and biologically evolving to become more transmissible over time.
Methodology: How Would They Work?
Reverse transcription could be used by a bad actor to alter the genetic structure of a target. Reverse transcription is the process by which RNA is converted back into DNA by an enzyme called reverse transcriptase. This process occurs naturally in retroviruses, which are a class of RNA viruses that can integrate their genetic material into the host's DNA.
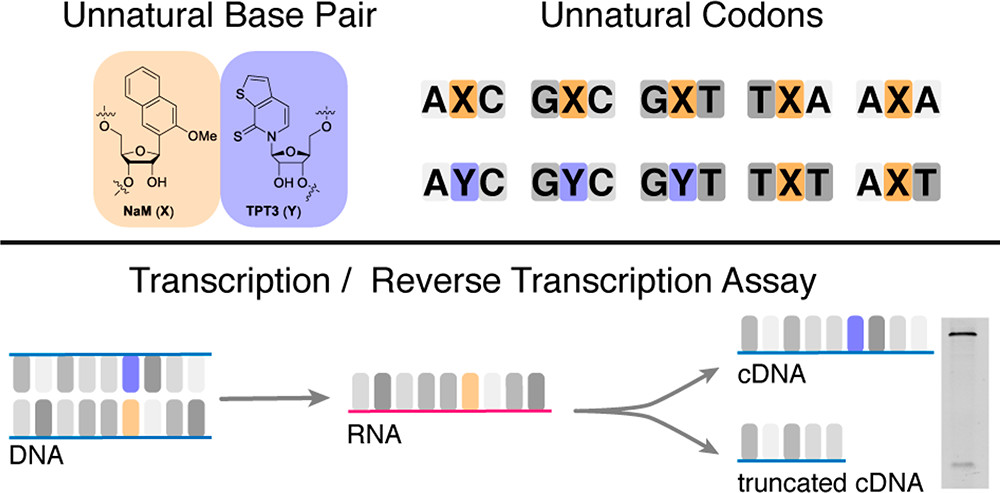
A bad actor could attempt to engineer a genetically modified virus that uses reverse transcription to deliver and integrate specific genetic alterations into the target population's cells.
Identify a genetic marker unique to the target population
Scientists would first need to identify a specific genetic marker that is unique or significantly more common in the target population. This could be a specific gene or a set of genes associated with a particular ethnic group or demographic.
Design a custom gene sequence
The antagonist would then need to create a custom gene sequence that, when integrated into the target's DNA, would cause the desired harmful effects. This could be achieved by designing a gene sequence that produces a toxic protein, disrupts normal cellular function, or induces a harmful immune response.
Creating a custom gene sequence that produces, for example, a toxic protein, involves several steps of genetic engineering. The specific details of this process can vary based on the type of toxin, the desired expression system, and other factors.
- Identify and Design the Toxic Protein: The first step is to identify a protein that has a toxic effect on cells when expressed. This could be a naturally occurring toxin, or a protein that interferes with cellular processes in a harmful way. Once the toxic protein has been identified, its gene sequence can be determined or designed.
- Gene Synthesis: Using techniques such as polymerase chain reaction (PCR) or gene synthesis, a piece of DNA encoding the toxic protein can be created. This synthetic gene would be designed to be compatible with the intended host organism, taking into account factors such as codon usage and promoter sequences.
- Cloning the Gene: The synthetic gene is then inserted into a plasmid, a small, circular piece of DNA that can replicate independently of chromosomal DNA. The insertion point is usually at a specific site within the plasmid known as a multiple cloning site (MCS).
- Transformation: The plasmid containing the synthetic gene is introduced into a host organism, typically bacteria, yeast, or mammalian cells in a lab setting. This process, known as transformation, can be accomplished via various methods including heat shock, electroporation, or chemical methods.
- Expression and Verification: Once the host organism has been transformed, it can be cultured under conditions that promote the expression of the toxic protein. The presence and function of the toxic protein can be confirmed using techniques such as Western blotting or assays that measure the protein's toxic effects.
Engineer a viral vector
The bad actor would need to choose a suitable viral vector, such as a modified retrovirus or another type of virus capable of integrating its genetic material into the host's genome. This virus would need to be engineered to specifically target cells containing the identified genetic marker and to deliver the custom gene sequence into those cells.
It might be possible to modify a weak common virus so that it behaves like a retrovirus, but it would be a complex and challenging process. Retroviruses are unique in their ability to convert their RNA genome into DNA and integrate it into the host cell's genome using the reverse transcriptase enzyme. This ability to integrate into the host genome is a key characteristic of retroviruses and the primary reason they have been used as vectors for gene therapy and other genetic manipulations.
To modify a weak common virus to behave like a retrovirus, several modifications would need to be made:
- Incorporation of reverse transcriptase: The weak common virus would need to be genetically modified to encode a functional reverse transcriptase enzyme, which would allow it to convert its RNA (if it's an RNA virus) or DNA (if it's a DNA virus) into a DNA molecule that could integrate into the host genome.
- Integration machinery: The virus would also need to be modified to include the necessary machinery for integrating its DNA into the host genome. In retroviruses, this function is typically performed by the integrase enzyme. The weak common virus would need to encode a functional integrase or a similar enzyme that can facilitate the integration process.
- Targeting specific cells: If the goal is to specifically target certain cells or populations, the virus would need to be engineered to recognize and bind to specific cell surface receptors that are unique to or more prevalent in the target population.
- Stability and safety: Modifying a weak common virus to behave like a retrovirus would require extensive genetic manipulation, which could potentially affect the stability and safety of the virus. Ensuring that the modified virus remains weak and does not cause unintended harm would be an important consideration.
Reverse transcription and integration
Once the viral vector infects a target cell, the custom gene sequence, in the form of RNA, would be reverse transcribed into DNA by the viral reverse transcriptase enzyme. This newly synthesized DNA would then be integrated into the host's genome, causing the intended genetic alteration.
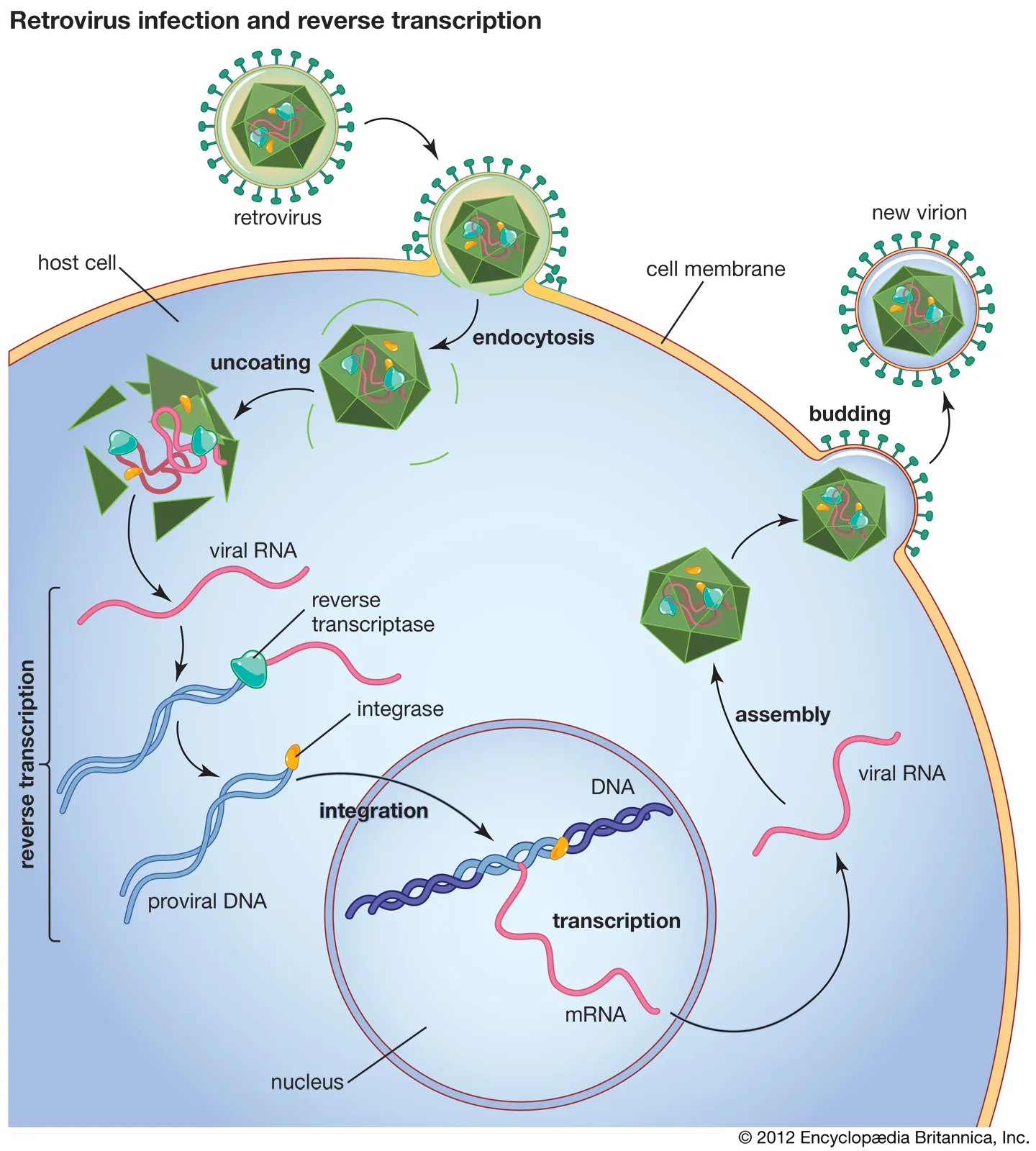
Reverse transcription is a process used by retroviruses, including HIV, to replicate their genetic material. Unlike most organisms that transcribe DNA into RNA, retroviruses reverse this process by transcribing RNA into DNA.
- Viral Entry: The process begins when a retrovirus infects a host cell. The virus attaches to a receptor on the host cell's surface, which allows the virus to fuse with the cell and release its RNA genome and enzymes, including reverse transcriptase, into the cell.
- RNA-DNA Hybridization: The reverse transcriptase enzyme binds to a specific site on the viral RNA. It uses this RNA as a template to start synthesizing a complementary strand of DNA.
- First Strand Synthesis: The reverse transcriptase continues to move along the RNA template, synthesizing the first DNA strand. This creates an RNA-DNA hybrid molecule.
- RNA Degradation: The RNA strand is then degraded by another activity of the reverse transcriptase called RNase H. This leaves the single-strand DNA molecule, also called the minus-strand DNA.
- Second Strand Synthesis: Next, reverse transcriptase synthesizes a second DNA strand complementary to the first strand, using the remaining RNA fragments as a primer. This generates a double-stranded DNA molecule, which is a replica of the original viral RNA genome.
- Integration: The double-stranded DNA molecule is then integrated into the host cell's DNA by another viral enzyme called integrase. Once integrated, the viral DNA can use the host's cellular machinery to produce new viruses.
Spread and manifestation of the effects
The engineered virus would need to be contagious, allowing it to spread within the target population. As more individuals become infected, the custom gene sequence would be integrated into their genomes, leading to the manifestation of harmful effects and potentially causing widespread illness or death.
How Would It Target A Group Selectively?
A population without the specific genetic marker targeted by the engineered virus would remain unaffected because the virus would be designed to specifically recognize and infect only cells containing that marker. This could be achieved by engineering the viral surface proteins to selectively bind to cell surface receptors that are either unique to, or more prevalent in, the target population.
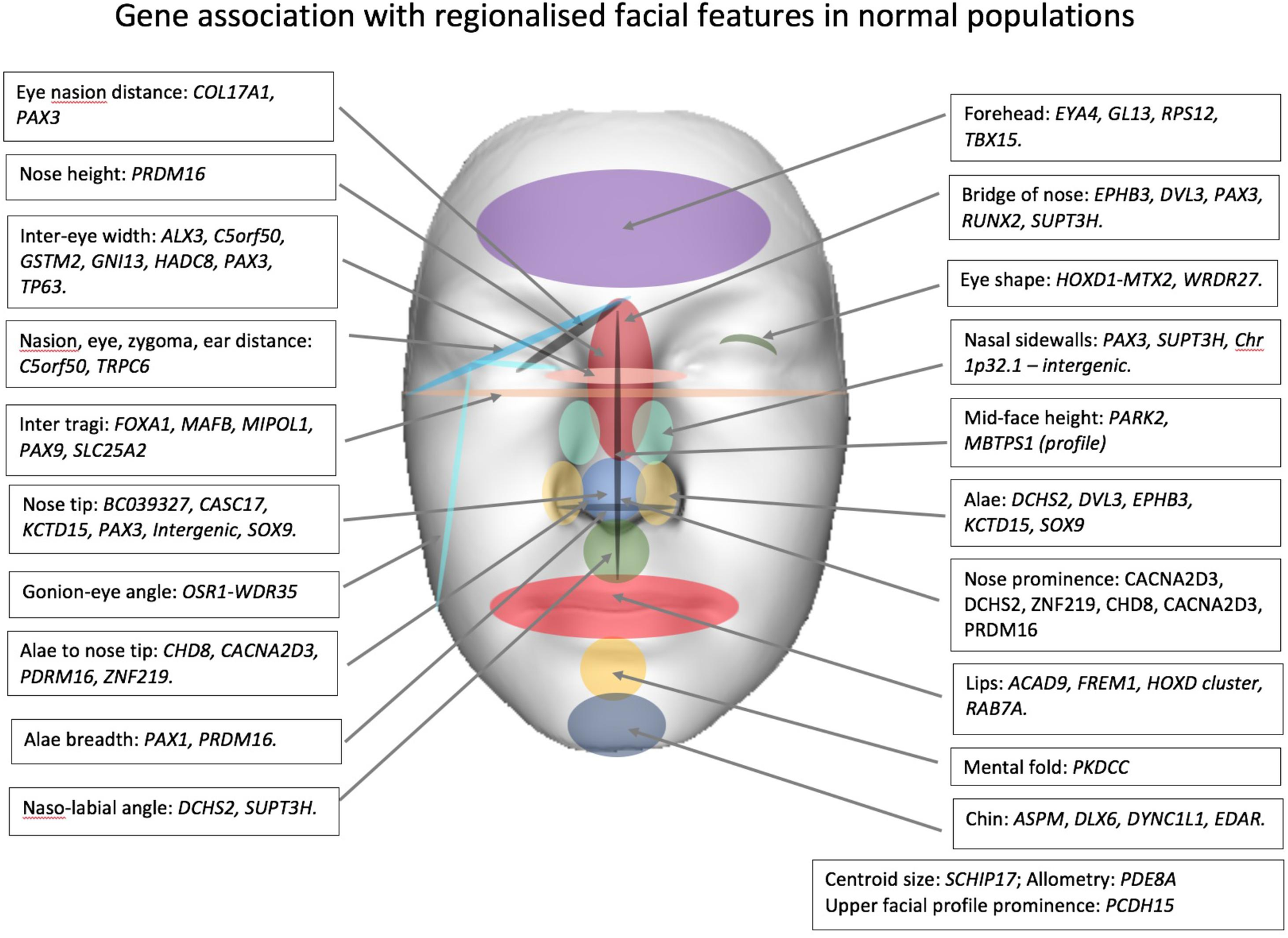
Genetic markers are DNA sequences that can be used to identify a specific location on a chromosome, and they can help researchers understand the genetic basis of various traits, including ancestry and susceptibility to certain diseases. Some genetic markers are more prevalent in specific populations due to factors like migration, genetic drift, and historical patterns of mating.
A few examples of genetic markers that are more common or unique to certain racial or ethnic populations:
- CCR5-Δ32: This is a small genetic mutation in the CCR5 gene that is more prevalent in individuals of European descent. This mutation provides some resistance to HIV infection.
- HbS: Hemoglobin S (HbS) is a variant of the beta-globin gene found in individuals with sickle cell anemia, a blood disorder that affects red blood cells. The HbS mutation is more common in people of African, Mediterranean, and Middle Eastern descent.
- ALDH22: This is a genetic variant of the aldehyde dehydrogenase 2 (ALDH2) gene, which is involved in alcohol metabolism. The ALDH22 allele is more prevalent in East Asian populations and is associated with reduced alcohol tolerance and increased risk of alcohol-related health issues.
- LCT: A genetic variant in the lactase (LCT) gene is associated with lactase persistence, which is the ability to digest lactose in adulthood. This trait is more common in populations with a history of dairy farming, such as those of Northern European descent.
It's important to emphasize that these genetic markers are not unique to these populations and that there is still considerable genetic diversity within each group. Targeting a single marker in a bioweapon would not uniformly affect all members of the intended population and could potentially impact others who possess the same marker but belong to a different demographic.
How Would It Kill?
There are multiple possibilities for a custom gene sequence that could cause harmful effects within 2-4 weeks or less after infection, along with potential viral vectors and their engineering to target vulnerable cells.
To engineer the viral vectors to target vulnerable cells, the virus's surface proteins could be modified to selectively bind to cell surface receptors that are either unique to or more prevalent in the target population.
This specificity could be achieved by incorporating a specific ligand or peptide sequence into the viral surface proteins, allowing the engineered virus to preferentially recognize and infect cells expressing the target receptor.
Targeting cells that play essential roles in multiple bodily functions would be the most effective strategy for lethality.
Hematopoietic Stem Cells
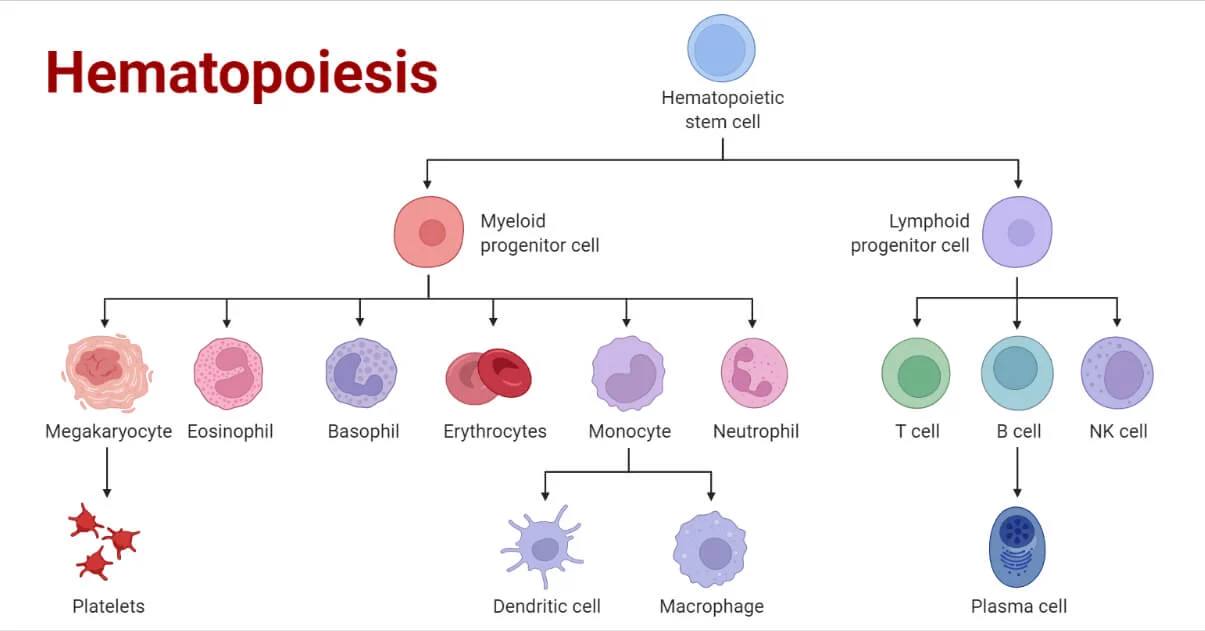
These cells reside in the bone marrow and give rise to all types of blood cells, including red blood cells (which carry oxygen), white blood cells (which fight infections), and platelets (which help in blood clotting).
Damaging these cells would lead to a decrease in red blood cells (causing anemia), white blood cells (causing immunodeficiency), and platelets (causing bleeding disorders). Symptoms could include fatigue, frequent infections, and easy bruising or bleeding.
This could become lethal over weeks to months as the body's immune system becomes progressively less able to fight off infections and other diseases.
Epithelial Cells in the Lungs
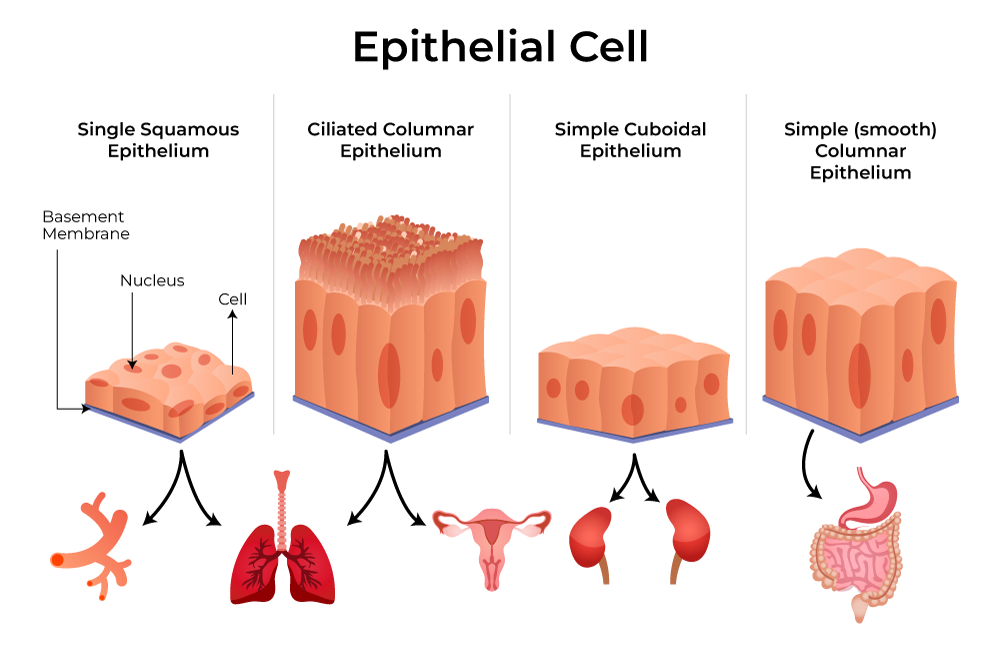
These cells line the airways and are involved in gas exchange, a crucial process for life. Attacking these cells could lead to severe respiratory distress, similar to conditions like pneumonia or acute respiratory distress syndrome (ARDS). See: https://journals.asm.org/doi/10.1128/JVI.00110-21
Symptoms would include severe shortness of breath, low oxygen levels, and respiratory failure. This could potentially be lethal within days to weeks.
Hepatocytes
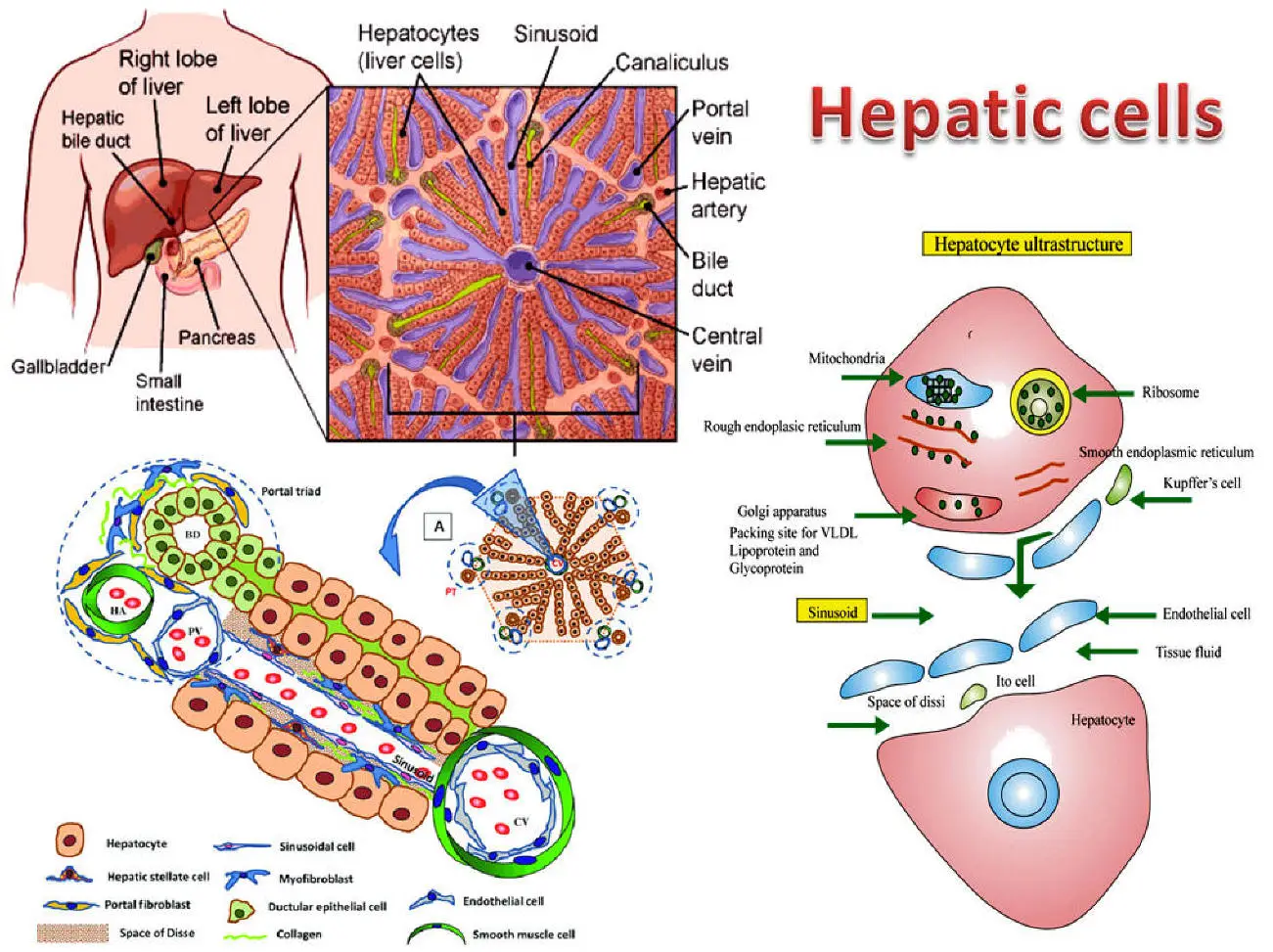
These are the primary cell types in the liver, an organ that plays a crucial role in metabolism, detoxification, and production of important proteins. These cells play a vital role in many bodily functions, including detoxification, protein synthesis, and digestion. If these cells were damaged, it could lead to liver failure.
Symptoms could include jaundice (yellowing of the skin and eyes), abdominal pain and swelling, nausea, and cognitive changes such as confusion or coma. Liver failure can be lethal within days to weeks.
Induction of cytokine storm (hypercytokinemia)
The gene sequence could be designed to overproduce specific pro-inflammatory cytokines, leading to a cytokine storm. A cytokine storm is an excessive immune response that can cause widespread inflammation, tissue damage, and potentially organ failure.
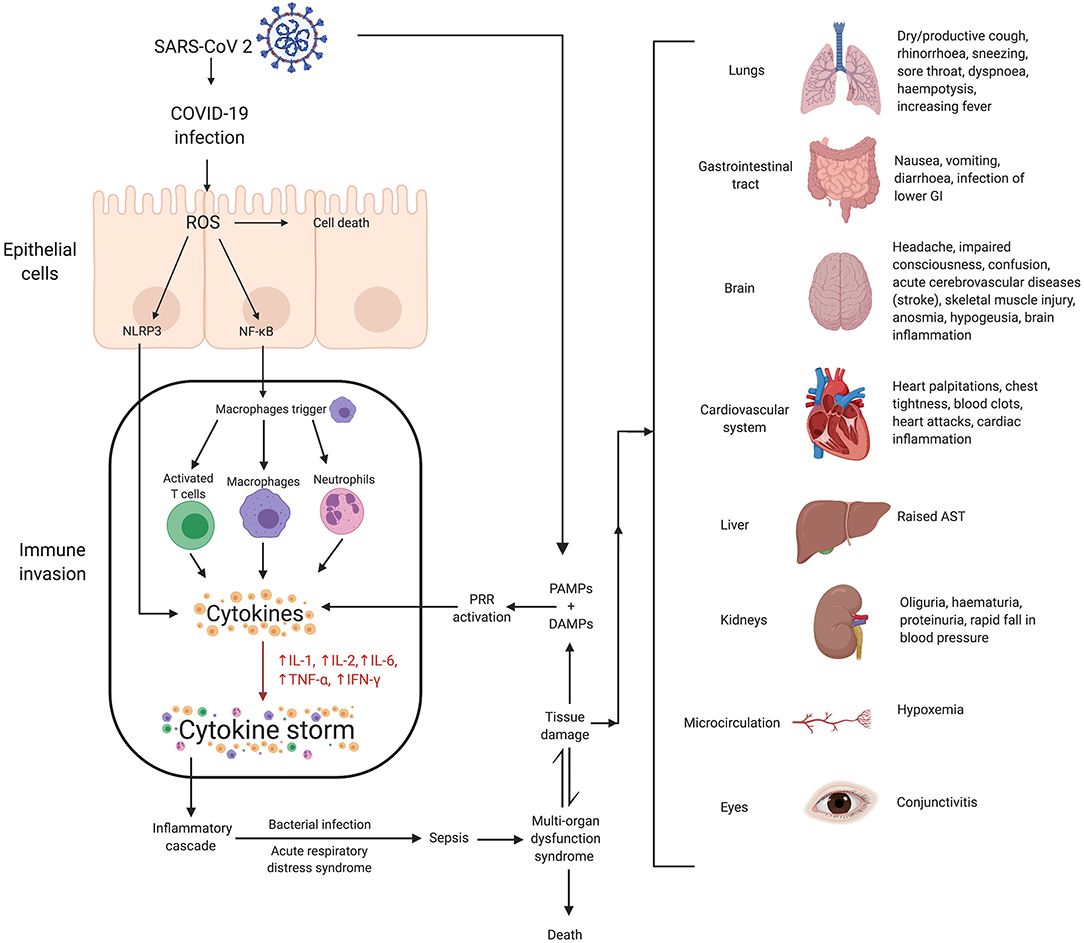
The body starts to attack its own cells. It can cause high fever, swelling and redness, extreme fatigue, and nausea. If not treated, it can lead to more severe symptoms, such as organ failure and death. This could occur rapidly, possibly within days to a week after the onset of symptoms.
Influenza virus or a modified adenovirus could be used as the vector. Both of these viruses are capable of respiratory transmission, which may facilitate rapid spread within the target population.
Disruption of cell cycle regulation
The gene sequence could be designed to disrupt normal cell cycle regulation, leading to uncontrolled cell growth and, eventually, the formation of tumors or rapid cell death.
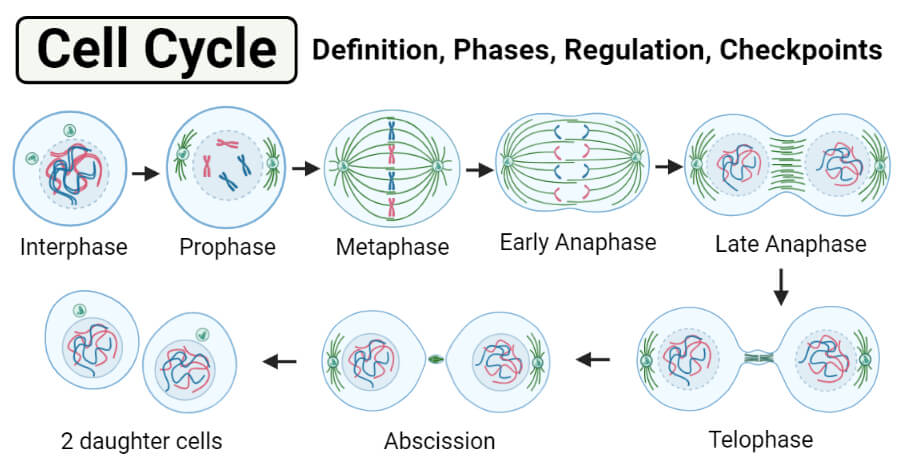
This would cause cells to proliferate uncontrollably, similar to cancer, or to die prematurely. Symptoms would depend on the cell types affected but could include weight loss, fatigue, pain, and organ dysfunction. This would likely be a slower process, potentially taking weeks to months to become lethal.
A modified retrovirus or lentivirus could be used as the vector. These viruses are capable of integrating their genetic material into the host genome, which would allow the harmful gene sequence to be expressed within the target cells.
- https://www.khanacademy.org/science/ap-biology/cell-communication-and-cell-cycle/regulation-of-cell-cycle/a/cell-cycle-regulators
- https://en.wikipedia.org/wiki/Lentivirus
Targeting cellular energy production
The gene sequence could be designed to interfere with cellular energy production by disrupting the function of mitochondria, the cell's energy-producing organelles. This disruption could lead to a rapid decline in cell function and ultimately cell death.
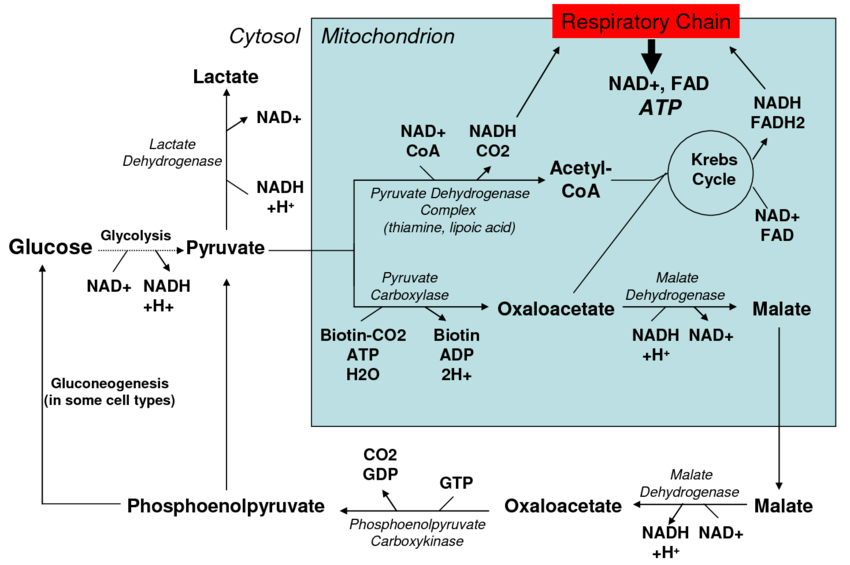
If the virus interferes with the cells' ability to produce energy (for example, by disrupting the function of the mitochondria), this could lead to a variety of symptoms, including fatigue, muscle weakness, and organ dysfunction. Depending on the severity of the disruption, death could occur within weeks.
A modified herpesvirus could be used as the vector. Herpesviruses can establish latent infections in a variety of cell types, which could facilitate the long-term persistence and expression of the harmful gene sequence.
- https://www.nature.com/scitable/topicpage/mitochondria-14053590/
- https://en.wikipedia.org/wiki/Herpesviridae
Could mRNA Be Misused To Achieve The Same Thing?
The misuse of mRNA technology in a harmful way would, in theory, follow a similar path to the misuse of viral vector technology, albeit with some key differences. mRNA technology, such as that used in some COVID-19 vaccines, delivers a small piece of mRNA into cells. This mRNA provides instructions for the cell's own machinery to produce a specific protein. In the case of the COVID-19 "vaccine", this protein is a piece of the SARS-CoV-2 virus, which triggers an immune response.
A bad actor would theoretically design an mRNA sequence that codes for a toxic protein that disrupts cellular functions, triggers an immune overreaction (similar to a cytokine storm), or interferes with crucial biological pathways. For example, a protein that inhibits the production of blood cells, impairs oxygen exchange in the lungs, or disrupts liver function.
The mRNA could be encapsulated in lipid nanoparticles, similar to the COVID-19 vaccines, to protect it from degradation and aid in delivery to cells.
They would need to find a way to deliver this mRNA preferentially to cells of the target demographic. This might involve attaching specific ligands to the lipid nanoparticles that bind to receptors more commonly expressed in the target demographic, or finding a way to co-opt naturally occurring physiological differences.
How could you prosecute?
The development, production, stockpiling, and use of bioweapons, including those targeting specific genetic markers, are prohibited under several international laws and treaties. Some of the key legal instruments that could be relevant in charging a person involved in creating and using such weapons include:
Biological and Toxin Weapons Convention (BTWC)
The BTWC, which entered into force in 1975, is an international treaty aimed at banning the development, production, and stockpiling of biological and toxin weapons. It prohibits the use of biological agents, including viruses, bacteria, and toxins, for any purpose other than peaceful or defensive reasons.
https://disarmament.unoda.org/biological-weapons/
The Geneva Protocol
The Protocol for the Prohibition of the Use in War of Asphyxiating, Poisonous or Other Gases, and of Bacteriological Methods of Warfare, commonly known as the Geneva Protocol, is an international treaty that prohibits the use of chemical and biological weapons in warfare. It was adopted in 1925 and entered into force in 1928.
International Humanitarian Law (IHL)
IHL, also known as the laws of war, governs the conduct of armed conflicts and seeks to protect individuals who are not, or are no longer, participating in hostilities. The use of bioweapons, including genetically-targeted ones, would likely be considered a violation of IHL, as such weapons would cause unnecessary suffering and have indiscriminate effects.
International Criminal Law
International criminal law encompasses a range of crimes that are considered to be of concern to the international community, such as war crimes, genocide, and crimes against humanity. Depending on the context and scale of the person's actions, they could be charged with one or more of these crimes in an international criminal tribunal, such as the International Criminal Court (ICC).
Further Reading & References
- "The potential impact of synthetic biology on the development of microbial bioweapons" by Giuseppe Novelli et al., published in Genetics and Molecular Biology (2006).
- "Gene therapy: implications for molecular bioweaponry" by Mark Wheelis, published in Journal of Medical Ethics (2002).
- "Biosecurity and Synthetic Biology: New Challenges for Dual Use Education" by Alexander Kelle, published in Biosecurity and Bioterrorism: Biodefense Strategy, Practice, and Science (2009).
- "Bioweapons and emerging infections: Recounting the threats" by Peter B. Jahrling et al., published in Proceedings of the National Academy of Sciences (2005).
- "Next-Generation Bioweapons: The Technology of Genetic Engineering Applied to Biowarfare and Bioterrorism" by Michael J. Ainscough, published in the Global Issues series.
- "The growing threat of biological weapons" by Anthony Tucker-Jones, published in Asian Affairs (2020).
- "Synthetic biology and bioweaponry" by Piers D. Millett, published in The Bridge on Synthetic Biology (2014).
- "The rapid development of synthetic biology and its impact on biosecurity" by James Revill and Catherine Jefferson, published in Biochemist (2014).
- "Current capabilities and future developments of biological agent-based weapons" by James Revill, published in Medicine, Conflict and Survival (2015).
- "Dual-use research and technological diffusion: reconsidering the bioterrorism threat spectrum" by Kathleen M. Vogel, published in PLoS Pathogens (2011).